The speedy approach used to tackle SARS-CoV-2 could change the future of vaccine science.
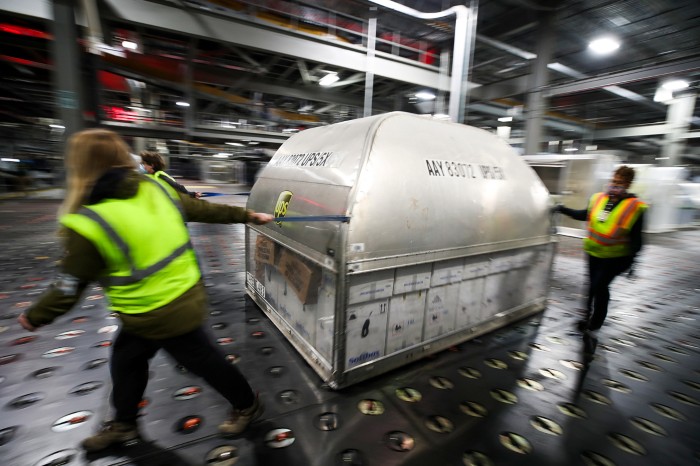
The first US shipments of the joint Pfizer–BioNTech COVID-19 vaccine were ready by mid-December.
When scientists began seeking a vaccine for the SARS-CoV-2 coronavirus in early 2020, they were careful not to promise quick success. The fastest any vaccine had previously been developed, from viral sampling to approval, was four years, for mumps in the 1960s. To hope for one even by the summer of 2021 seemed highly optimistic.
But by the start of December, the developers of several vaccines had announced excellent results in large trials, with more showing promise. And on 2 December, a vaccine made by drug giant Pfizer with German biotech firm BioNTech, became the first fully-tested immunization to be approved for emergency use.
That speed of advance “challenges our whole paradigm of what is possible in vaccine development”, says Natalie Dean, a biostatistician at the University of Florida in Gainesville. It’s tempting to hope that other vaccines might now be made on a comparable timescale. These are sorely needed: diseases such as malaria, tuberculosis and pneumonia together kill millions of people a year, and researchers anticipate further lethal pandemics, too.
The COVID-19 experience will almost certainly change the future of vaccine science, says Dan Barouch, director of the Center for Virology and Vaccine Research at Harvard Medical School in Boston, Massachusetts. “It shows how fast vaccine development can proceed when there is a true global emergency and sufficient resources,” he says. New ways of making vaccines, such as by using messenger RNA (mRNA), have been validated by the COVID-19 response, he adds. “It has shown that the development process can be accelerated substantially without compromising on safety.”
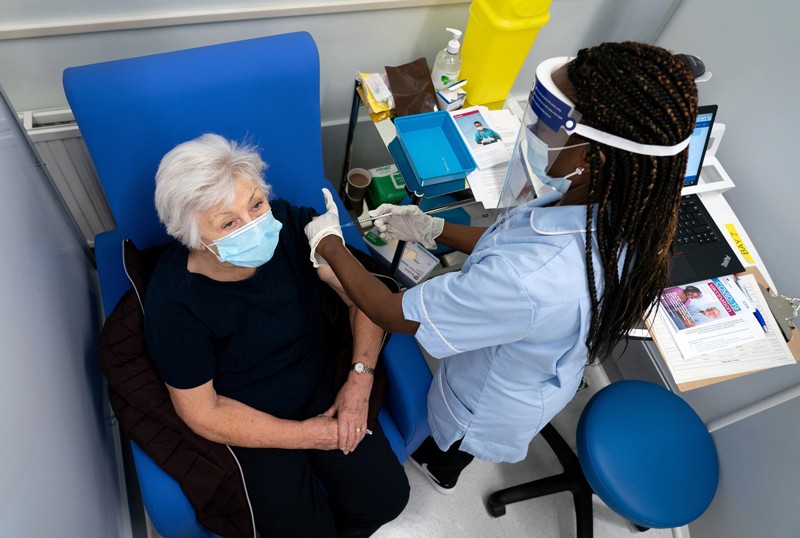
The first UK injections of a fully tested COVID-19 vaccine were given in early December.
The world was able to develop COVID-19 vaccines so quickly because of years of previous research on related viruses and faster ways to manufacture vaccines, enormous funding that allowed firms to run multiple trials in parallel, and regulators moving more quickly than normal. Some of those factors might translate to other vaccine efforts, particularly speedier manufacturing platforms.
But there’s no guarantee. To repeat such rapid success will require similar massive funding for development, which is likely to come only if there is a comparable sense of social and political urgency. It will depend, too, on the nature of the pathogen. With SARS-CoV-2, a virus that mutates relatively slowly and that happens to belong to a well-studied family, scientists might — strange as it sounds — have got lucky.
Years of advance research
The research that helped to develop vaccines against the new coronavirus didn’t start in January. For years, researchers had been paying attention to related coronaviruses, which cause SARS (severe acute respiratory syndrome) and MERS (Middle East respiratory syndrome), and some had been working on new kinds of vaccine — an effort that has now paid off spectacularly.
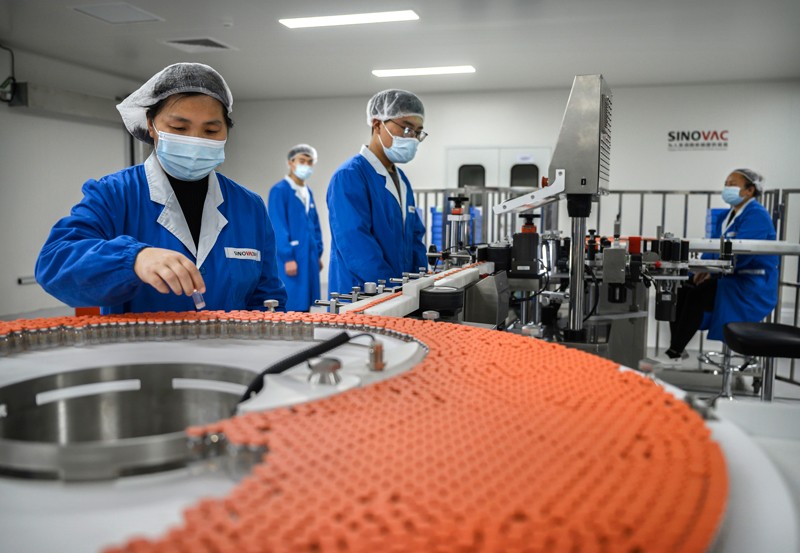
Vials of Sinovac Biotech’s COVID-19 vaccine on a production line in Beijing.
Conventional vaccines contain viral proteins or disabled forms of the virus itself, which stimulate the body’s immune defences against infection by a live virus. But the first two COVID-19 vaccines for which efficacy was announced in large-scale (phase III) clinical trials used just a string of mRNA inside a lipid coat. The mRNA encodes a key protein of SARS-CoV-2; once the mRNA gets inside our cells, our bodies produce this protein. That acts as the antigen — the foreign molecule that triggers an immune response. The vaccines made by Pfizer and BioNTech and by the US pharmaceutical company Moderna both use mRNA that encodes the spike protein, which docks to human cell membranes and allows the coronavirus to invade the cell.
“A lot went into the mRNA platform that we have today,” says immunologist Akiko Iwasaki at the Yale School of Medicine in New Haven, Connecticut, who has worked on nucleic-acid vaccines — those based on lengths of DNA or RNA — for more than two decades. The basic research on DNA vaccines began at least 25 years ago, and RNA vaccines have benefited from 10–15 years of strong research, she says, some aimed at developing cancer vaccines. The approach has matured just at the right time; five years ago, the RNA technology would not have been ready.
For instance, researchers at the US National Institute of Allergy and Infectious Diseases (NIAID) in Bethesda, Maryland, knew from their research on MERS and SARS that it was best to tune the RNA sequence to stabilize the resulting spike protein in the form it adopts before it docks with a host cell. “If you can trap it in its original pre-fusion state, it becomes a much better vaccine antigen,” says Barney Graham, deputy director of NIAID’s vaccine research centre. That work gave the NIAID team, which worked with Moderna, a head start once SARS-CoV-2 was sequenced in January. “The fact that people had been paying close attention to coronaviruses really allowed this whole process to accelerate,” says Dean.
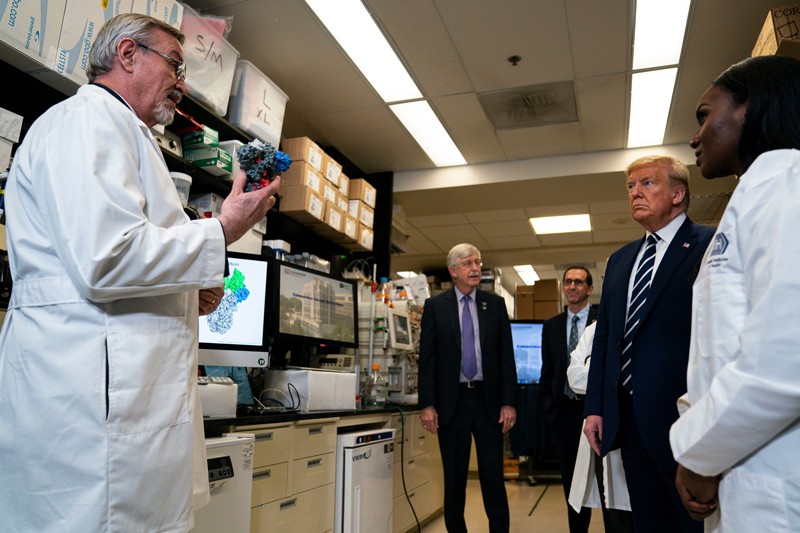
Barney Graham, deputy director of the NIAID Vaccine Research Center (VRC; left), talks to US President Donald Trump (second from right) during a visit in March. VRC scientists at the centre, co-led by researcher Kizzmekia Corbett (far right), created RNA sequences for COVID-19 vaccines in early January. Also pictured are NIH director Francis Collins (second from left) and VRC director John Mascola (third from left).
The third vaccine to show efficacy in phase III clinical trials in November, made by the pharmaceutical firm AstraZeneca with the University of Oxford, UK, does not use mRNA. Instead, a viral vector (or carrier) holds extra genetic material that codes for the SARS-CoV-2 spike protein. This, too, benefited from years of research to select the vector; in this case, the firm chose a modified form of adenovirus isolated from chimpanzee stool. Advances in conventional vaccines such as these have also come from research on SARS, MERS, Ebola and malaria, says Beate Kampmann, director of the Vaccine Centre at the London School of Hygiene & Tropical Medicine, and such approaches remain cheaper than using mRNA.
Vaccine researchers were fortunate with SARS-CoV-2 in many respects, says Iwasaki. The virus doesn’t mutate a lot or have effective strategies for foiling the human immune system, she says, unlike HIV, herpes or even influenza. The herpes virus, by contrast, has more evasion capability — it actively blocks antibodies from binding, which makes it harder to find an effective agent against it. And the fast mutation of flu viruses requires a different vaccine formulation for every flu season.
Supercharged with funding
The slowest part of vaccine development isn’t finding candidate treatments, but testing them. This often takes years (see ‘Vaccine innovation’), with companies running efficacy and safety tests on animals and then in humans. Human testing requires three phases that involve increasing numbers of people and proportionately escalating costs. The COVID-19 vaccines went through the same trials, but the billions poured into the process made it possible for companies to take financial risks by running some tests at the same time (see ‘A vaccine in a year’).
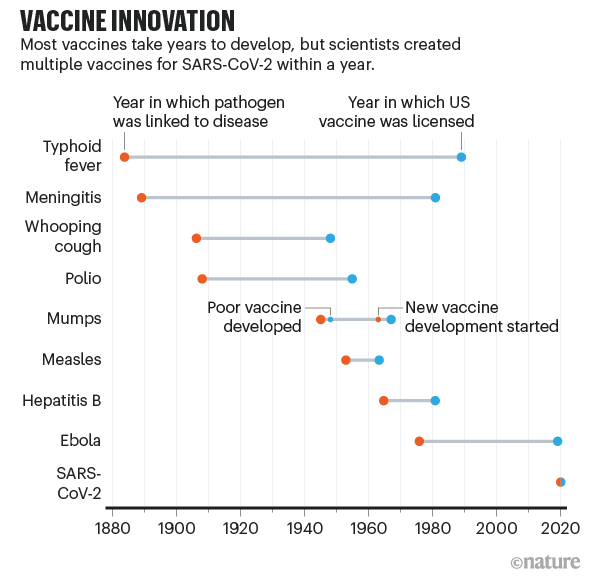
Sources: Our World in Data; Nature analysis
With large sums given to vaccine firms by public funders and private philanthropists, “they could do preclinical and phase I, II and III trials, as well as manufacturing, in parallel instead of sequentially”, says Rino Rappuoli, chief scientist at GlaxoSmithKline’s vaccines division in Siena, Italy. This meant that companies could gamble on starting large-scale testing and manufacturing of candidates that might not work out. “It was totally de-risking the entire development process,” says Kampmann.
The vaccine science would not have produced such fast results without this funding, she says. “It didn’t happen with Ebola, which was devastating communities in Africa [in 2014–16]” — and Ebola vaccines accordingly took longer to develop. The money only materialized this time because all countries, including wealthy ones, faced economic devastation: suggesting that the development of future vaccines, including for existing diseases such as malaria, will not be as speedy. “Unless you put in the money, there’s no way to accelerate,” says Rappuoli.
Virologist Peter Hotez at Baylor College of Medicine in Houston, Texas, suggests that large pharmaceutical companies might have been motivated not just by the desire to stop the pandemic, but also by the opportunity for governments to fund their research and development. With public investment of around US$10 billion, the US Operation Warp Speed vaccine programme “represents the largest government stimulus package the pharma companies have ever seen”, says Hotez.
The impetus didn’t all come from the urgency of the COVID-19 pandemic itself. Previous infectious and lethal viruses have motivated the creation of national and global infrastructures that can promote faster vaccine development. The Ebola and Zika outbreaks saw the beginning of better global coordination in how to respond to an infectious-disease crisis, Graham says. “If SARS in 2002 had spread like this, we wouldn’t have had the vaccine technology or the coordinated systems, and we’d have had a much more difficult time,” he says.
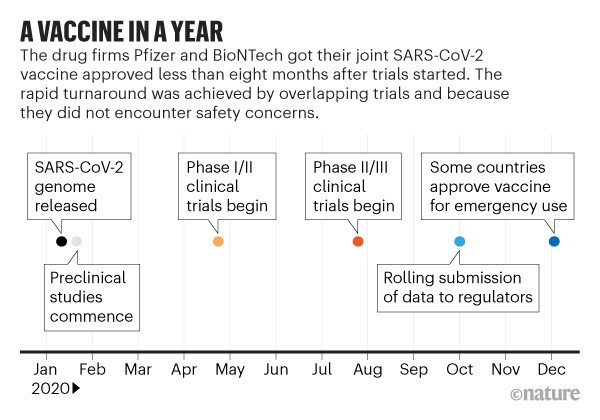
Sources: BioNTech/Pfizer; Nature analysis
In particular, the Coalition for Epidemic Preparedness Innovations (CEPI) was launched in 2017; its goal is to create the technological infrastructure needed for rapid and affordable development of vaccines against several of the viruses known to have epidemic potential, including MERS, Ebola and Zika. CEPI has partly funded work on SARS-CoV-2 vaccines, including that by Moderna and at Oxford.
In the final stages of trials, it helped that COVID-19 was everywhere because firms need infections to show that vaccines work. It’s hard to run efficacy trials when the diseases themselves aren’t prevalent — especially, says Dean, in cases such as MERS, for which outbreaks of disease were patchy, with peaks in some areas and low infection rates in others.
The COVID-19 experience might also prompt a regulatory rethink. Although there has been no relaxing of the stringent criteria for vaccine approval, the first candidates are mostly being approved under emergency-use regulations. These are faster but require companies to conduct follow-up surveys to look for side effects and continuing efficacy. National regulators also swapped information on COVID-19 vaccine trials under the auspices of a global body called the International Coalition of Medicines Regulatory Authorities, set up in 2012. It has aimed to reach consensus on issues such as the best end-points for vaccine trials, and how to harmonize the monitoring of side effects as vaccines are rolled out (see also Nature 588, 195; 2020).
Benefits for other vaccines
The COVID-19 pandemic should see some permanent changes in vaccine development. For a start, it might establish the use of mRNA vaccines — which hadn’t previously been approved for general use in people — as a speedy approach for other diseases. “This technology is revolutionizing vaccinology,” says Kampmann. Candidate mRNA vaccines can be chemically synthesized in a few days, in contrast to the more complicated biotechnology involved in producing proteins in cells. “The technology lends itself to the nimble plug-and-play approach that will be required to respond to [future] pandemics,” Kampmann says.
What’s more, “RNA simplifies the manufacturing a lot,” says Rappuoli. “You can use the same facility to make RNA for different diseases. That decreases the investment required.” Companies should also be ramping up their manufacturing capacities because they still have to make vaccines for measles, polio and other diseases even as they produce COVID-19 immunizations. That could help to meet demand in future.
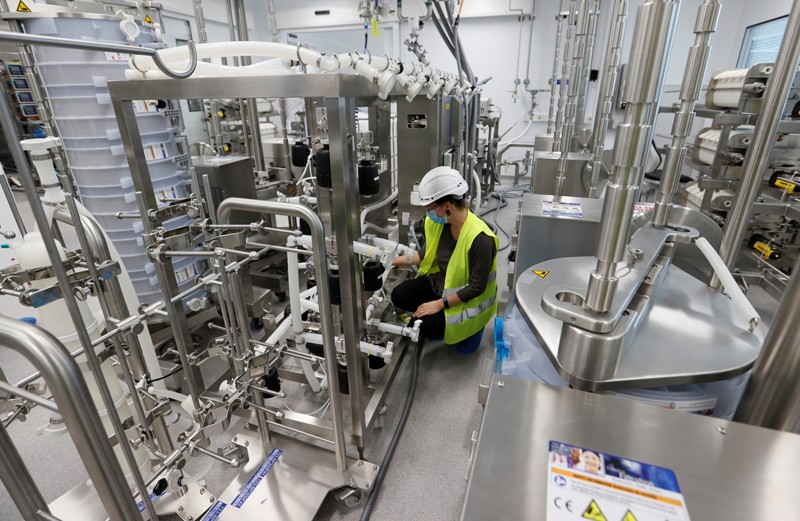
Moderna’s mRNA COVID-19 vaccine will be made by the biotech firm Lonza at these facilities in Visp, Switzerland.
The large clinical trials for COVID-19 vaccines, and others in development, should provide data that are more widely useful for understanding immune responses, says Hotez. “Given all the different technologies, and detailed information collected on clinical volunteer demographics, antibody and cellular responses, we might learn as much or more from human vaccine responses this year than in previous decades. Human vaccinology could make a quantum leap.”
Still, other vaccines can probably only be developed at a comparable speed when infection levels are high — making it possible to run massive trials relatively quickly — and with huge amounts of funding. And other viruses might be harder to target than SARS-CoV-2 turned out to be.
That’s why we need to know more about all families of viruses, say researchers. There are at least 24 other virus families that can infect humans, says Graham. Rather than waiting to sink resources into fighting the next virus that pops up, money would be better spent now setting up systems to monitor all these viruses and to generate data on prototype infections in each of these families, he says.
In other words, no amount of money will help without a solid platform of basic science to build on. The extraordinary success of the COVID-19 vaccines “is a good example of what science can do very quickly”, says Iwasaki, “but it didn’t happen overnight.”
No comments:
Post a Comment